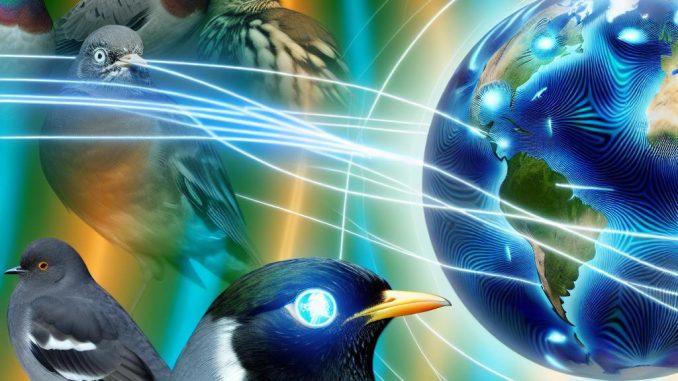
Understanding Avian Magnetic Perception
Birds have long fascinated scientists with their extraordinary ability to navigate Earth’s magnetic field. This remarkable skill enables them to undertake long migratory journeys with astounding precision. Recent research has unveiled the underlying biological mechanisms that enable birds to perceive magnetic fields, providing insight into this extraordinary capability.
The Role of Cryptochromes
Central to the avian capacity to detect magnetic fields are proteins known as cryptochromes. Located in the cells of birds’ eyes, cryptochromes are a type of flavoprotein sensitive to blue light. When they are exposed to certain wavelengths of light, these proteins undergo specific chemical reactions that produce radical pairs—pairs of molecules with unpaired electrons.
It is hypothesized that Earth’s magnetic field can influence the behavior of these radical pairs. As a result, birds have the ability to detect changes in the concentration of these radicals due to the orientation of the molecules in relation to the magnetic field. This allows them to perceive directional information in a way that is not yet fully understood by science.
The Role of Retinal Neurons
Researchers believe that changes in radical pairs provide certain visual cues within a bird’s retina, likely manifesting as unique patterns or shifting colors superimposed on their normal sight. This capacity has the potential to transform visual information into a practical navigational tool. Neuronal pathways then interpret these visual signals, enhancing the birds’ ability to align themselves with magnetic lines and aiding their ability to successfully migrate.
In this model, the retina not only senses changes but also transmits information to the brain for processing. This detection integrates seamlessly with the other visual cues birds receive from their environment, such as landmarks and celestial bodies, to form a comprehensive navigational strategy.
Related Studies
Numerous studies have supported the involvement of cryptochromes in magnetoreception. Experiments that involve modifying these proteins in certain bird species have shown changes or impairments in their navigational abilities, reinforcing the proposed role of these molecules. The link between cryptochrome function and magnetoreception has deepened scientific understanding of the sophisticated biological processes underlying avian navigation.
For instance, researchers have conducted experiments introducing mutations in the cryptochrome genes of birds to observe changes in navigational behavior. The outcomes consistently point toward a direct correlation between the cryptochromes’ structural integrity and the birds’ ability to successfully orient themselves in their migratory patterns. This strong correlation has continually emphasized the significance of these proteins in the birds’ natural compass system.
Quantum Biology and Magnetoreception
The interaction between cryptochromes and Earth’s magnetic field offers an insightful glimpse into the burgeoning field of quantum biology. This area of study explores how quantum mechanics, which is traditionally associated with physics, applies to biological processes. In the context of avian magnetoreception, the notion that quantum events occur within radical pairs to produce biologically significant responses is gaining interest.
Researchers are delving into how the principles of quantum mechanics may govern biological phenomena like magnetoreception. The radical pairs created within cryptochromes behave according to quantum rules, suggesting that biological entities can engage with environmental cues at a fundamentally quantum level. This interaction might be one of the earliest examples of how organisms leverage quantum principles for survival, potentially opening new frontiers in the comprehension of life’s complexity.
The radical pair model, a focus within quantum biology, postulates that light-induced electron transfers within cryptochromes can be influenced by weak magnetic interactions. This model implies that these influences, otherwise too subtle to affect classical chemical processes, may significantly affect the dynamics of electron spins and thus relay directionality information to the organism.
Ongoing Research
While significant advancements have been made in understanding bird magnetoreception, the exact nature of this phenomenon eludes complete understanding. Current research aims to elucidate the molecular and genetic foundations of this capability further, allowing scientists to probe deeper into how birds and possibly other animals navigate using magnetic fields.
Emerging studies continue to explore the possibility that other sensory modalities, acting in concert, contribute to magnetic orientation. Genetic studies are also being expanded to identify potential mutations or variations in cryptochrome genes across bird species to understand their implications on navigation skills.
The efforts in research not only focus on the biological and quantum realms but also pave the way for technological innovation. By understanding how cryptochromes participate in navigational strategies, engineers and technologists are inspired to develop new classes of sensors and devices potentially aiding human navigation without reliance on existing GPS technologies.
In conclusion, the science behind why birds can perceive magnetic fields illustrates a remarkable confluence of biology and physics. As research advances, our understanding of these mechanisms could not only illuminate concepts of animal navigation but also serve as a blueprint for pioneering technological advancements and enhance our comprehension of quantum biological processes.
Environmental and Technological Implications
Beyond understanding the fundamental science, there are broader implications of avian magnetoreception in environmental conservation and technology. Comprehending how birds interact with magnetic fields can inform conservation strategies, ensuring migratory pathways remain viable in the face of increasing human infrastructure developments.
In technology, insights gleaned from studying avian magnetoreception might foster novel approaches to designing sensors and navigation systems, especially for environments where traditional systems like GPS might be inadequate or unreliable. For instance, the concept of biomimicry—leveraging natural processes to inspire engineering solutions—could take inspiration from birds to create energy-efficient navigation tools.
As research into avian magnetoreception continues, it promises profound impacts beyond academic circles, potentially affecting numerous aspects of human life and the biodiversity we strive to protect. Understanding these birds’ unique mechanisms continues to spark curiosity and drive innovation, highlighting the intricate beauty of natural processes shaped by both biology and physics.